How bacteriophages could revolutionize treatment of fracture related infections
BY DR KYLE SCHWESER
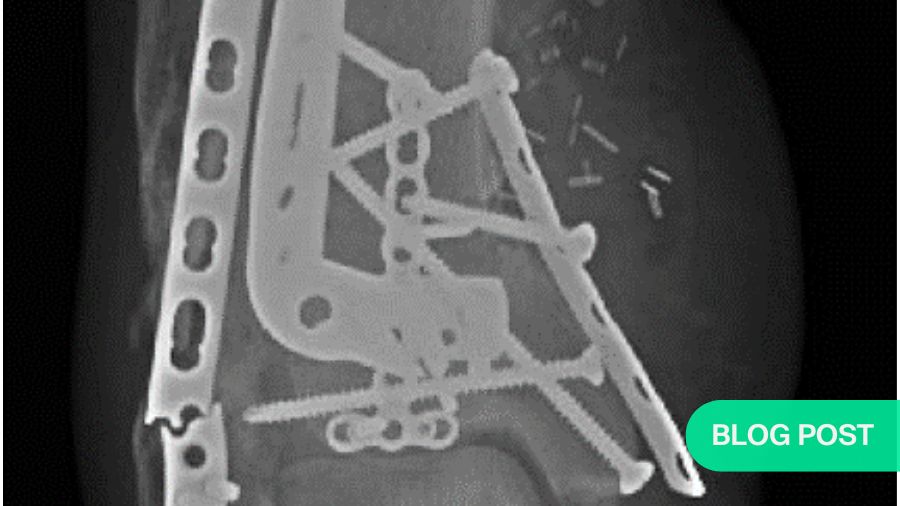
Fracture-related Infections (FRIs) present a challenging problem for both orthopedic surgeons and the patients who develop them. They occur with a greater frequency than is probably appreciated by most trauma surgeons, occurring in approximately 20% of all trauma cases. While this percentage may be disproportionate based on extremity location, it still holds true that 1 in every 5 trauma cases develops an infection. Treatment has evolved very little over the years, following some variation of an algorithm generally consisting of irrigation and debridement, differing levels of hardware exchange/retention, and antibiotic use. Beyond these traditional treatment options for FRIs, bacteriophages may have the greatest potential to help us.
Kyle Schweser’s study on “Treatment of Acute Fracture-Related Infections Utilizing Bacteriophage Therapy” won gold medal in the 2023 AO Trauma Research Olympiad and the 2023 Basic Science Chairman’s Award from the Orthopaedic Trauma Association (OTA).
While it’s difficult to generalize the success rates following the treatment of FRIs secondary to the differing characteristics of fracture morphology, location, soft tissue status, and patient comorbidities, it seems that union can be achieved 70–89% of the time. However, if we measure the success rate based on long term recurrence risk and hardware retention, the success rates fall. Long term recurrence rates seem to vary between 9–58% and retention of implants is only around 30–40%. That means that if we are measuring success by limiting secondary surgeries and eradication of infection, then we are not doing as good a job as we think.
While focusing on the numbers associated with success and failure is important, and easy to do, they do not convey the burden these infections place on the patients. The devastating effects financially, socially, physically, and mentally these infections have on patients cannot be overstated. Medical costs for FRIs are 6.5x more than noninfectious cases, and this does not even consider the loss of wages and productivity the patient experiences during a now unexpected and prolonged recovery course
The difficult management of FRIs is likely multifactorial (small colony variants, bacterial invasion of canaliculi, etc.), however, a major contributor is biofilm. Biofilm is notoriously difficult to eradicate, and if even a small percentage of biofilm remains on the implant, bone, or soft tissue, an infection can return. This, along with other known and unknown factors, likely contributes to infections returning decades after “successful” management of an FRI. The eradication of biofilm is paramount if we are to eradicate an infection and improve our long-term success rates in the treatment of FRIs. However, to do this, we need to think beyond the current, traditionally available, treatment options for FRIs. This is where bacteriophages have the greatest potential to help us.
What are bacteriophages?
Bacteriophages (phages) can be thought of (very simplistically) in the same way that we think of viruses for humans. Phages are viruses for bacteria, and they have likely evolved alongside bacteria to control bacterial populations. In fact, some estimates are that for every bacterial cell, there are 10 bacteriophages. This would make phages one of, if not the most, abundant organisms on earth. Phages infect bacterial cells the same way that virus infection our cells.
A very basic summarization of their mechanism is: Attachment to the cell, injection of genetic material, replication of bacteriophages, lysis of the cell, and release of phages. These released phages then go on to “infect” other bacterial cells until either the infection is eradicated, or a steady state of bacterial replication and bacteriophage infection occurs.
They also offer several advantages: They do not affect eukaryotic cells, they are bactericidal, toxicity is limited to bacterial byproducts, there is minimal disruption of normal flora, narrow potential for inducing resistance, no cross-resistance with antibiotics, and biofilm clearance. However, to really understand why current strategies struggle, and how phages can help us, we need an understanding of biofilm.
Understanding the complexity of biofilm and why antibiotics struggle to work
Early on in my training, I pictured biofilm as an inert slime mold that bacteria could hide in. It was a thick substance that prevented antibiotics from entering and allowed bacteria to replicate until they eventually made their way out. However, this could not be further from the truth. Biofilm should be thought of more as a living organism that is coordinated and sophisticated in both its form and function. I’ll do my best to convey the complexity of biofilm in this short narrative. Please excuse the brevity.
While this is supposed to be a discussion on bacteriopahges, it is critically important to understand biofilm so we can better understand how to treat it. First, biofilm isn’t one solid slime mold where bacteria freely float around. It is actually made up of numerous microcolonies of bacteria tightly packed together and surrounded by a hydrogel layer, keeping them independent of the surrounding environment and other microcolonies. These tightly packed communities of bacteria communicate through quorum sensing, enabling rapid transmission of information and genetic material, thus allowing for swift adaptation in response to the surrounding environment.
These colonies are then stacked on top of each other in layers and communicate via channels, which allows for one microcolony to share information with other microcolonies in a coordinated effort to produce more extracellular polymeric substances (EPS/biofilm), efflux channels, transition into planktonic bacteria, produce lytic enzymes to break down the biofilm for release, etc. This means that the release of bacteria from the biofilm isn’t a random event. It is coordinated in response to the changes occurring outside of the biofilm that make propagation of bacteria viable or hostile.
However, none of this means much if antibiotics were effective against biofilm, but they aren’t. This is because biofilm offers multiple different layers of protection against antibiotic therapy, however, we will focus on three big ones: Resistance at the surface, resistance within the microenvironment, and resistance at a cellular level.
Resistance at the surface
- There is a myth that antibiotics can’t penetrate biofilm, many can. It’s just that they aren’t effective once they do. The first part of neutering antibiotics occurs at the surface of the biofilm. The outer layer of biofilm limits the penetration of antibiotics. This limited ability to penetrate the surface means that bactericidal or bacteriostatic concentrations can never be achieved. For educational purposes, think of it like needing 10 molecules of antibiotics to penetrate the biofilm every second to reach therapeutic concentrations, however, the surface layer only allows 2 molecules every second. This means that concentrations will never reach a necessary level to be effective. However, why is the concentration never reached, as opposed to, taking longer to reach it? This has to do with the environment, and what lives within the environment, that they encounter upon penetration.
Resistance within the microenvironment
- Once the antibiotics penetrate the surface layer, they then encounter the layered microcolonies of bacteria. The hydrogel surrounding these colonies offers another line of defense limiting the antibiotics ability to access the bacteria. These colonies are also stacked so tightly, that diffusion between them is limited. This further slows/limits the penetration of antibiotics and decreases their ability to reach appropriate concentrations.
As the antibiotics move deeper into the biofilm, they encounter an increasingly hostile environment. The environment becomes more anaerobic and acidic which directly antagonizes the antibiotic activity of drugs like tobramycin, ciprofloxacin, and aminoglycosides, with deeper levels of the biofilm being so acidic outside of the microcolonies that most antibiotics are rendered ineffective. Why then, doesn’t the slowed diffusion allow for certain superficial layers of the biofilm to reach therapeutic concentrations?
Resistance at a cellular level
- As the antibiotics penetrate and begin to encounter the microcolonies, some can penetrate and gain access to the bacteria within. However, because they can’t do so at high enough concentrations, this allows for the bacteria to adapt to the antibiotic. This is where being so tightly packed is advantageous. Once a bacterial cell upregulates its efflux pumps, produces beta-lactamases, changes their membrane, etc. in response to an antibiotic, it immediately begins communicating via quorum sensing. This allows for rapid adaptation of all the bacteria within the microcolony.
The resistant microcolony then communicates via channels to other microcolonies telling them to adapt. This means that a microcolony of bacteria can develop resistance to an antibiotic before the antibiotic has even reached its intended target.
As antibiotics move deeper into the biofilm, they encounter highly resistant phenotypes of bacteria secondary to multiple factors, including slowed metabolic activity and hardening in response to the changing environment within the biofilm. In fact, they have shown that a planktonic form of bacteria can be sensitive to an antibiotic, but once this same bacterium is taken from the biofilm in its sessile form, it is now resistant to the same antibiotic. They also encounter bacterial forms called “persister cells.” These are dormant bacteria that have entered a spore-like state that are capable of withstanding extreme environments. These “spores” are not a different genetic makeup than the rest of the bacteria present in the biofilm, just in a different state. This state is completely resistant to antibiotics because they are metabolically inactive. This means that even if an antibiotic were able to eradicate all the microcolonies present within the biofilm, as soon as the antibiotics were removed, the persister cells would become active again, changing back into their sessile or planktonic forms.
Now that you have a birds-eye view of the structure and function of biofilm, we can discuss how bacteriophage specifically address each of these problems.
Phages vs Biofilm—what we need to know
Phages also have difficulty penetrating the thick structure of biofilm, and there are several factors beyond this talk that can contribute to biofilm phage resistance, however, phages do have a mechanism for biofilm penetration. Phages produce a variety of enzymes whose sole function is to breakdown EPS. Depolymerases, lysins, proteases, etc are all secreted by bacteriophages to breakdown EPS, essentially dissolving the biofilm and creating holes for further penetration. This can also be effective against the hydrogel surrounding the microcolonies. The lysins and nucleases produced by the phages also cleave cell walls and bacterial DNA. These enzymes allow for rapid, deep penetration of phages into the biofilm and direct access to bacterial cells. Thus, circumventing some of the antibiotic challenges of resistance at the surface. This also changes the microenvironment, increasing oxygenation and decreasing acidity as the biofilm becomes more porous and loses waste biproducts that generate acidic environments.
Once they have access to the bacteria, they attach to receptor binding proteins present on the cell surface. At first, the attachment is temporary, however, once a phage is irreversibly bound to a bacterium, lysis will eventually occur. As the phages break down and infect bacteria, the ability of the bacteria to generate more EPS and undergo replication is hindered, meaning that less biofilm and bacteria are produced. This step has no link to antibiotic resistance. As long as the bacteria is susceptible to the phage, infection of the bacterial cell will occur. While there are some papers that examine optimal phage concentrations, this has more to do with the fact that the interaction between the bacteria and bacteriophage is random (the more phages present, the higher the chance of an interaction occurring) and the effects of passive vs active phage therapy (active—you add phages, passive—relying on phage replication within bacteria), and less to do with phage effectiveness. Theoretically, one phage infecting one bacteria is all that is necessary to begin to breakdown the microcolonies and biofilm, meaning that the effectiveness is not dependent on reaching some level of therapeutic concentrations.
Persister cells are problematic, even for bacteriophages, however, they are not immune. While a persister cell may be metabolically inactive, they still contain receptor binding proteins on the surface that phages can interact with. While we know that phages can infect persister cells, it’s not yet clear if they can drive the persister cell to become metabolically active, or if the phage genetic material lies dormant until the persister cell returns to its metabolically active state. Regardless, once a phage has passed on its genetic material, the persister cell will eventually undergo lysis.
While the resistance mechanisms for antibiotics can still present a challenge for phages (plus additional phage resistance mechanisms), none of them are completely effective and phages have developed to be a much more effective solution to bacteria and biofilm when compared to antibiotics.
Challenges in translating bacteriophages use into clinical setting
If bacteriophages are so great at clearing infection, then why aren’t we using them more? This answer is complicated and multifaceted. The benefits of phages are well known, and they have been extensively studied in Europe, especially in Soviet Russia and Georgia. In fact, you can obtain an oral cocktail of phages for the treatment of diarrhea in some European countries. The United States military has a phage research facility, and the NIH has awarded millions for phage related research.
However, this research has mostly focused on either non-orthopaedic infections or centered on osteomyelitis and chronic implant related infections. There is very little information available regarding how a phage affects fracture healing or its efficacy in managing acute infections. Optimal phage dosing and administration is also relatively unknown, and problems exist with current methods in terms of translation to a clinical setting, especially in the United States healthcare system. Phages are fragile, and have few natural defenses, so systemic administration is challenging as they are readily cleared from the circulatory system. Oral administration can be effective, but the acidic environment of the stomach is incredibly hostile to phages. Local administration seems to be effective at managing infections, but the logistics of administration in terms of method, frequency, and timing are challenging for clinical translation.
Resistance can occur towards phages, as well. While biofilm penetration is a function of bacteriophages, that does not mean it isn’t without its challenges, especially in chronic infections. Bacteria and biofilm have evolved alongside phages and can produce several substances like amyloid fibers which can inhibit phage penetration. CRISPR-associated gene expression, phage proteases, and the production of receptors that decrease phage adsorption are observed in bacterial populations. Bacteria are highly adaptable and can adjust rapidly to changes in their surroundings, so it makes sense that they would adapt to combat phages. However, some data suggests that phages can adapt more rapidly than bacteria, making this an evolutionary cat and mouse game. This adjustment likely won’t occur rapidly enough to affect acute treatment for infections; however, our knowledge is limited in this regard.
Another challenging aspect of phage application for the treatment of infection in the United States is its classification by the FDA. In Europe, phages are classified as biologics while in the US they are classified as a drug. This makes the mass production and use of phages difficult, as phage therapy tends to be patient specific, with variations in bacterial susceptibilities between patients. Current use in the US is limited to compassionate use only, and very few companies exist that contain a large enough library of phages who are willing to contribute to the clinical care of a patient. These regulatory challenges will continue to persist until we know more about things like storage, dosing, sterility, etc.
Phages offer a promising solution for treating drug resistant infection in orthopedic fractures
Phages offer a promising alternative to infection control, especially considering the concern with multi-drug resistant infections, however, more data is needed. While research in this field has been around for decades, the quality of older data can be suspect and the translatability of it to orthopaedic infections, especially acute infections, is limited.
From an orthopaedic standpoint, we need a better understanding of how phages interact with our immune system (variability exists in the literature from a robust adaptive immune response to no response), how we should dose phages (concentrations, volume, delivery mechanisms, etc.), synergistic effects with antibiotics and other infection management practices, and how they affect things like fracture healing to name a few. More translational research is needed to allow for robust clinical trials. A few clinical trials are underway, including the management of diabetic wounds and periprosthetic joint infections, and case reports are available on the treatment of chronic osteomyelitis, however, human data is extremely sparce. Other fields of study involving phages, such as the use of their degradative enzymes for biofilm, are also promising and may help avoid some of the regulatory and administrative issues phages present.
Regardless of the current issues around the mass translation of phages to clinical practice and the sparce human data available (especially concerning fracture infection care), phages offer a promising solution to a difficult problem and the orthopedic community should continue to study and advance this field of research as we strive to combat an ever-increasing threat of MDR bacteria.
About the author:
You might also be interested in:
AO Trauma Research Olympiad
The event connects AO Trauma regions and promotes research on a global level. Take part or sign up for the next AO Trauma Research Olympiad.
AO PEER
The AO Program for Education and Excellence in Research is a knowledge platform designed for health care professionals who want to learn and improve their clinical research skills.
AO Trauma Fellowship Program
Discover the unique opportunities to advance in your training and deepen your expertise.
Clinical Research Fellowships
The three-month, hands-on coaching program with AO ITC is for surgeons who want in-depth training in clinical research.