Computer-assisted surgery: Evolution and basic concepts
Preview
As the field of robotics evolves, so too does our willingness to welcome technology into traditionally human-dominated realms. Computer-assisted surgery (CAS) is available for the modern operating room and is constantly being refined to enhance functionality, precision, and cost-effectiveness. Part 1 chronicles some of the evolution of CAS, looking at terminology and basic concepts.
Through the ages, humans have been fascinated with the idea of automated devices that serve our needs. Consider Hephaestus’ mechanical servants in Greek mythology [1]. We have been entranced by the potential of machines for millennia [2,3]. Now, our capabilities are catching up to our imaginings: take a look at robotics lab Boston Dynamics’ newest commercial robot, Spot® [4].
Advancements in technology, combined with interdisciplinary team collaboration, is propelling the integration of innovations in materials, computing, and medicine to bring a technological revolution to the operating room (OR) across all surgical disciplines.
What is computer-assisted surgery?
Computer-assisted surgery (CAS) is a umbrella term that encompasses different kinds of technologies that are used to [1]: perform surgical procedures, in part or in their entirety [2]; guide or navigate during surgery [3]; plan surgeries [4]; train less experienced surgeons [5]; and create patient-specific instruments (PSI) [5–7]. It is sometimes referred to as computer-aided surgery, computer-assisted intervention, surgical robots, image-guided surgery, or surgical navigation, depending on what the technology does [5, 7, 8].
CAS helps orthopedic surgeons perform surgery with increased precision and reproducibility, which is believed to have a positive impact on clinical and functional outcomes. There are two categories of CAS: robotic-assisted surgery, where a motor moves the technology, and general CAS (usually navigation systems), where the surgeon physically moves the technology [9].
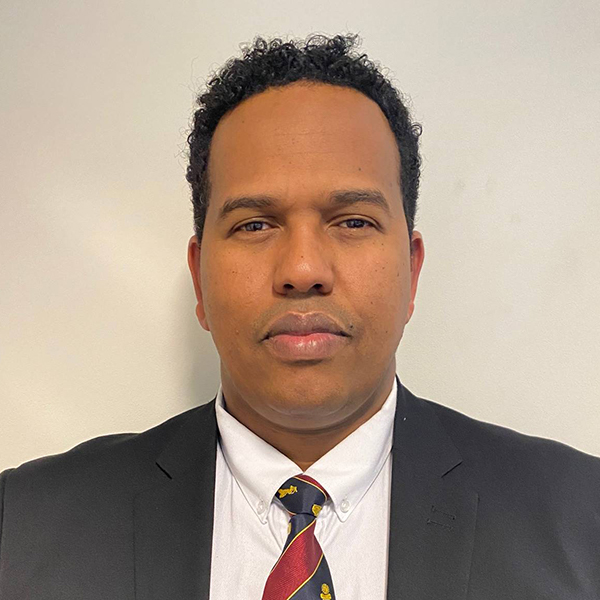
Ahmed Magan
University College London Hospital
London, United Kingdom
Ahmed Magan, Trauma and Orthopedic Surgeon with University College London Hospital NHS Foundation Trust, UK, notes that robotic surgery in particular “has revolutionized surgical practice—from planning through to the execution of the operation. It is easy to learn and the results are reproducible.”
It all started with the brain
The pioneering work of CAS was in the field of neurosurgery in the early 1900s. Two British academics working at University College London Hospital, Sir Victory Horsley (professor of neurosurgery and a neuroscientist) and British physiologist, Robert Clarke, collaborated on the development of a stereotactic apparatus for locating lesions in the brain in 1908.
It essentially involved attaching the cranium to the “Horsley-Clarke Apparatus” and inserting a probe into an area of interest with some degree of accuracy. Their work was based on three-dimensional (3D) Cartesian geometry of a monkey brain [10]. It is no wonder that their invention lacked precision and required further work.
Applications of CAS
Over time, CAS systems have been developed for use in a wide range of surgical disciplines. An indicator of the growth of the field is that in 1999, only 14 articles indexed in PubMed included “computer-assisted surgery” or “robotic surgery” in their titles or abstracts, while by 2019, 1,027 articles were published.
Neurosurgery was the first field to employ CAS [8, 11, 12], and the technology has expanded to support myriad surgical interventions. Here are a few examples:
- Total hip arthroplasty (THA): Robotic THA was found to improve acetabular implant positioning and reduce dislocations compared to manual THA [13, 14].
- Partial and total knee arthroplasty (TKA): Using navigation in TKA was associated with higher clinical accuracy in implant placement [15] and robot-assisted TKAs also improved implant positioning [16].
- Osteotomies: Using 3D-planned patient-specific instrumentation (PSI) and navigation in high tibial open wedge valgus-producing osteotomies resulted in accurately corrected mechanical leg axis [17].
- Tumors: Minimally invasive robotic hepatectomy for liver tumors has been shown to be “safe and feasible” [18]. Intraoperative computer-assisted navigation and 3D PSI printing facilitated a successful surgical resection of metastatic acetabular osteosarcoma, ultimately preserving the patient’s hip stability and providing better quality of life for two palliative years [19].
- Neurosurgery: Robot-assisted drainage of thalamic hemorrhages improved patients’ prognoses and was associated with reduced cases of pneumonia and renal dysfunction [20].
- Spine surgery: A study of 18 patients that received navigation-assisted surgery for a primary spine tumor indicated that it was beneficial in the resection of tumors due to more accurate screw placement and fewer complications [21].
- Dental implants: Compared with a novice freehand implant placement group, novices using navigation were able to achieve implant placement in mandible models with an accuracy similar to that of experienced professionals [22].
In relation to orthopedics, Ahmed Magan highlights that, “In terms of contributions, computer-assisted surgery has been a real game-changer in partial and TKA. With this procedure, the outcome is related to the alignment, balance, and soft tissue preservation and there is technology available to help surgeons achieve these factors in more reliable, repeatable ways.
“However, there are barriers to this technology being widely adopted and available in all ORs such as, added operative time, being limited to implants specific to the system, resistance to new technology training for theater staff and cost implications.”
Read the full article with your AO login
- Classification of CAS systems
- Navigation
- Image-based navigation
- Imageless navigation
- Patient-specific instrumentation (PSI)
- Optimized positioning systemTM
- Virtual and augmented reality
- Artificial intelligence
- Open vs closed CAS robotic systems
- Path of development
- Robotics in surgery
- Early surgical robots
- Conclusion
- References
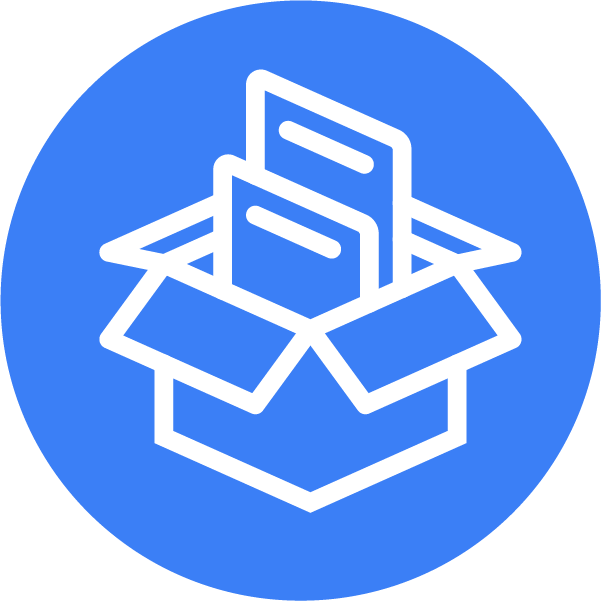
Additional AO resources on this topic
Access videos, tools, and other assets to learn more about this topic.
- Video: Robotics and Other Smart Tools for Hip and Knee Replacement
- Further reading: The development and validation of a robotic system for sacroiliac luxation/fracture reduction and fixation
- Upcoming events: AO Recon Course finder
Contributing experts
This series of articles was created with the support of the following specialists (in alphabetical order):
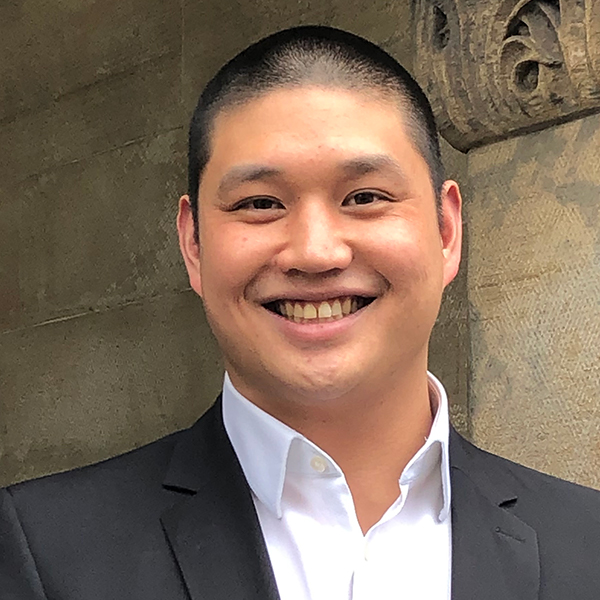
Justin Chang
University College London Hospital
London, United Kingdom
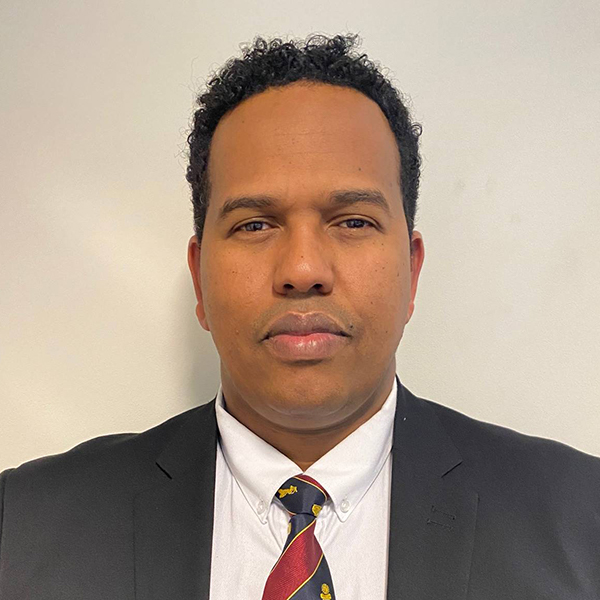
Ahmed Magan
University College London Hospital
London, United Kingdom
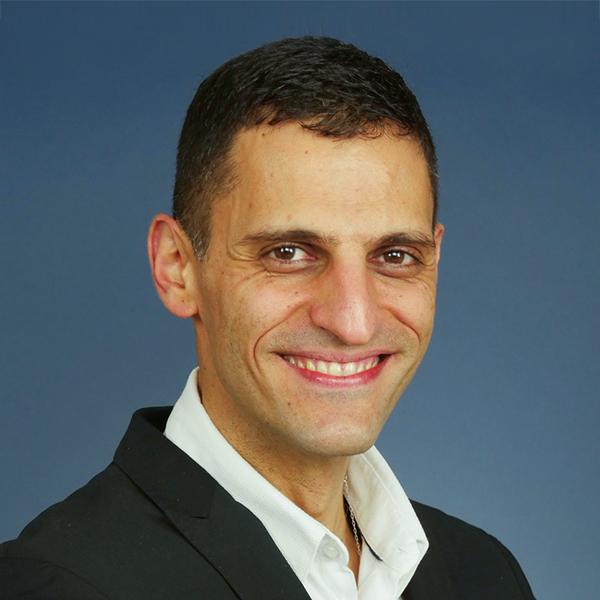
Mark Roussot
University College London Hospital
London, United Kingdom
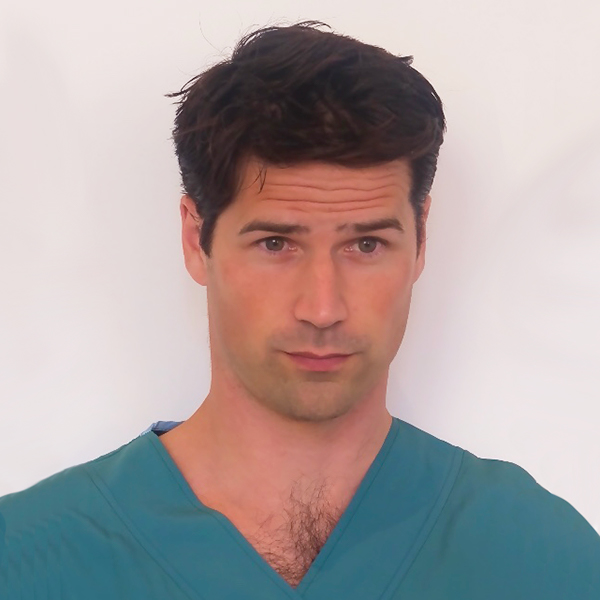
Georges Vles
University Hospitals Leuven
Leuven, Belgium
This issue was created by Word+Vision Media Productions, Switzerland
References
- Marino MV, Shabat G, Gulotta G, et al. From Illusion to Reality: A Brief History of Robotic Surgery. Surg Innov. 2018 Jun;25(3):291–296.
- Needham J. Science and Civilisation in China: Volume 2, History of Scientific Thought. Cambridge University Press; 1991.
- Yates DR, Vaessen C, Roupret M. From Leonardo to da Vinci: the history of robot-assisted surgery in urology. BJU Int. 2011 Dec;108(11):1708–1713; discussion 1714.
- Boston Dynamics. SPOT®. https://www.bostondynamics.com/spot. Published 2019. Updated November 5, 2019. Accessed April 10, 2020.
- Kazanzides P. Robots for Orthopaedic Joint Reconstruction. In: Faust R, ed. Robotics in Surgery: History, Current and Future Applications. New York: Nova Science Publishers Inc.; 2006:61–94.
- Jackson DW, Simon TM. History of computer-assisted orthopedic surgery (CAOS) in sports medicine. Sports Med Arthrosc Rev. 2008 Jun;16(2):62–66.
- Picard F, Deakin AH, Riches PE, et al. Computer assisted orthopaedic surgery: Past, present and future. Med Eng Phys. 2019 Oct;72:55–65.
- Wikipedia. Computer-assisted surgery. https://en.wikipedia.org/wiki/Computer-assisted_surgery. Published 2020. Updated March 24, 2020. Accessed April 10, 2020.
- Davies B. A review of robotics in surgery. Proc Inst Mech Eng H. 2000 214(1):129–140.
- Horsley V, Clarke R. The structure and functions of the cerebellum examined by a new method. Brain. 1908 31(1):45–124.
- Jenny JY. [The history and development of computer assisted orthopaedic surgery]. Orthopade. 2006 Oct;35(10):1038–1042.
- Moore E. Robotic surgery. Encyclopædia Britannica, inc. https://www.britannica.com/science/robotic-surgery. Published November 23, 2018. Accessed April 10, 2020.
- Illgen R, Bukowski B, Abiola R, et al. Robotic-Assisted Total Hip Arthroplasty: Outcomes at Minimum Two-Year Follow-Up. Surg Technol Int. 2017 July;25(30):365–372.
- Kayani B, Konan S, Ayuob A, et al. The current role of robotics in total hip arthroplasty. EFORT Open Rev. 2019 Nov;4(11):618–625.
- Selvanayagam R, Kumar V, Malhotra R, et al. A prospective randomized study comparing navigation versus conventional total knee arthroplasty. J Orthop Surg (Hong Kong). 2019 May–Aug;27(2):2309499019848079.
- Bell SW, Anthony I, Jones B, et al. Improved Accuracy of Component Positioning with Robotic-Assisted Unicompartmental Knee Arthroplasty: Data from a Prospective, Randomized Controlled Study. J Bone Joint Surg Am. 2016 Apr 20;98(8):627–635.
- Fucentese SF, Meier P, Jud L, et al. Accuracy of 3D-planned patient specific instrumentation in high tibial open wedge valgisation osteotomy. J Exp Orthop. 2020 Feb 27;7(1):7.
- Sucandy I, Schlosser S, Bourdeau T, et al. Robotic hepatectomy for benign and malignant liver tumors. J Robot Surg. 2020 Feb;14(1):75–80.
- Heunis JC, Cheah JW, Sabnis AJ, et al. Use of three-dimensional printing and intraoperative navigation in the surgical resection of metastatic acetabular osteosarcoma. BMJ Case Rep. 2019 Sep 30;12(9).
- Wang Y, Jin H, Gong S, et al. Efficacy Analysis of Robot-Assisted Minimally Invasive Surgery for Small-Volume Spontaneous Thalamic Hemorrhage. World Neurosurg. 2019 Nov;131:e543–e549.
- Ando K, Kobayashi K, Machino M, et al. Computed tomography-based navigation system-assisted surgery for primary spine tumor. J Clin Neurosci. 2019 May;63:22–26.
- Jorba-García A, Figueiredo R, González-Barnadas A, et al. Accuracy and the role of experience in dynamic computer guided dental implant surgery: An in-vitro study. Med Oral Patol Oral Cir Bucal. 2019 Jan 1;24(1):e76–e83.
- Picard F, Moody J, DiGioia A, et al. Clinical Classification of CAOS Systems. In: DiGioia A, Jaramaz B, Picard F, et al, eds. Computer and Robotic Assisted Hip and Knee Surgery. Oxford: Oxford University Press; 2004:43–48.
- Taylor R. Robotics in Orthopedic Surgery. In: Nolte R, Ganz R, eds. Computer assisted orthopedic Surgery (CAOS). Boston: Hogrefe & Huber Publishers; 1998:35–41.
- Lane T. A short history of robotic surgery. Ann R Coll Surg Engl. 2018 May;100(6_sup):5–7.
- Picard F, Moody J, Jaramaz B, et al. A Classification Proposal for Computer-Assisted Knee Systems. 2000; Berlin, Heidelberg.
- Davies B. A brief review of robotics in surgery. Proc Instn Mech Engrs. 1999 24:129–140.
- Levinson K. Robotic Assisted Surgery. Electrical and Computer Engineering Design Handbook. 2015. https://sites.tufts.edu/eeseniordesignhandbook/. Accessed April 14, 2020.
- Chang JD, Kim IS, Bhardwaj AM, et al. The Evolution of Computer-Assisted Total Hip Arthroplasty and Relevant Applications. Hip Pelvis. 2017 Mar;29(1):1–14.
- Okamura AM. Haptic feedback in robot-assisted minimally invasive surgery. Curr Opin Urol. 2009 19(1):102–107.
- Zamorano L, Li Q, Rhiew R. Robotic Applications in Neurosurgery. In: Faust R, ed. Robotics in Surgery: History, Current and Future Applications. New York: Nova Science Publishers Inc; 2006:147–172.
- Choi PJ, Oskouian RJ, Tubbs RS. Telesurgery: Past, Present, and Future. Cureus. 2018 May 31;10(5):e2716.
- Marescaux J, Leroy J, Gagner M, et al. Transatlantic robot-assisted telesurgery. Nature. 2001 Sept;413(6854):379–380.
- George EI, Brand TC, LaPorta A, et al. Origins of Robotic Surgery: From Skepticism to Standard of Care. JSLS. 2018 Oct–Dec;22(4).
- Wikipedia. Remote surgery. Wikipedia. https://en.wikipedia.org/wiki/Remote_surgery. Published 2019. Updated November 25, 2019. Accessed April 18, 2020.
- Marescaux J, Leroy J, Rubino F, et al. Transcontinental robot-assisted remote telesurgery: feasibility and potential applications. Ann Surg. 2002 Apr;235(4):487–492.
- Victor J, Hoste D. Image-based computer-assisted total knee arthroplasty leads to lower variability in coronal alignment. Clin Orthop Relat Res. 2004 Nov;(428):131–139.
- Lang JE, Mannava S, Floyd AJ, et al. Robotic systems in orthopaedic surgery. J Bone Joint Surg Br. 2011 Oct;93(10):1296–1299.
- Sugano N. Computer-assisted orthopaedic surgery and robotic surgery in total hip arthroplasty. Clin Orthop Surg. 2013 Mar;5(1):1–9.
- Venkatesan M, Mahadevan D, Ashford RU. Computer-assisted navigation in knee arthroplasty: a critical appraisal. J Knee Surg. 2013 Oct;26(5):357–361.
- Sikorski J, Chauhan S. Computer-assisted orthopaedic surgery: Do we need CAOS? The Journal of bone and joint surgery British volume. 2003 05/01;85:319–323.
- Mezger U, Jendrewski C, Bartels M. Navigation in surgery. Langenbecks Arch Surg. 2013 Apr;398(4):501–514.
- Zheng G, Nolte LP. Computer-Assisted Orthopedic Surgery: Current State and Future Perspective. Front Surg. 2015 2:66.
- Kubicek J, Tomanec F, Cerny M, et al. Recent Trends, Technical Concepts and Components of Computer-Assisted Orthopedic Surgery Systems: A Comprehensive Review. Sensors (Basel). 2019 Nov 27;19(23).
- Hacihaliloglu I. Ultrasound imaging and segmentation of bone surfaces: A review. Technology (Singap World Sci). 2017 Jun;5(2):74–80.
- US Food & Drug Administration. Computed Tomography (CT): Risks and Benefits. https://www.fda.gov/radiation-emitting-products/medical-x-ray-imaging/computed-tomography-ct#3. Published 2019. Updated June 14, 2019. Accessed April 18, 2020.
- Chen TK, Abolmaesumi P, Pichora DR, et al. A system for ultrasound-guided computer-assisted orthopaedic surgery. Comput Aided Surg. 2005 Sep-Nov;10(5-6):281–292.
- US Food & Drug Administration. MRI (Magnetic Resonance Imaging) Benefits and Risks. https://www.fda.gov/radiation-emitting-products/mri-magnetic-resonance-imaging/benefits-and-risks. Published 2017. Updated September 12, 2017. Accessed April 18, 2020.
- Beitzel J, Ahmadi SA, Karamalis A, et al. Ultrasound bone detection using patient-specific CT prior. Conf Proc IEEE Eng Med Biol Soc. 2012 2012:2664–2667.
- Wein W, Karamalis A, Baumgartner A, et al. Automatic bone detection and soft tissue aware ultrasound-CT registration for computer-aided orthopedic surgery. Int J Comput Assist Radiol Surg. 2015 Jun;10(6):971–979.
- Akins R, Abdelgawad AA, Kanlic EM. Computer navigation in orthopedic trauma: safer surgeries with less irradiation and more precision. J Surg Orthop Adv. 2012 Winter;21(4):187–197.
- Thakkar SC, Thakkar RS, Sirisreetreerux N, et al. 2D versus 3D fluoroscopy-based navigation in posterior pelvic fixation: review of the literature on current technology. Int J Comput Assist Radiol Surg. 2017 Jan;12(1):69–76.
- Saragaglia D, Rubens-Duval B, Gaillot J, et al. Total knee arthroplasties from the origin to navigation: history, rationale, indications. Int Orthop. 2019 Mar;43(3):597–604.
- Brin YS, Nikolaou VS, Joseph L, et al. Imageless computer assisted versus conventional total knee replacement. A Bayesian meta-analysis of 23 comparative studies. Int Orthop. 2011 Mar;35(3):331–339.
- Liu Z, Gao Y, Cai L. Imageless navigation versus traditional method in total hip arthroplasty: A meta-analysis. Int J Surg. 2015 Sep;21:122–127.
- Kowal J, Langlotz F, Nolte L. Basics of Computer-Assisted Orthopaedic Surgery. In: Stiehl J, Konermann W, Haaker R, et al., eds. Navigation and MIS in Orthopedic Surgery. Germany: Springer Medizin Verlag Heidelberg; 2007:2–8.
- Docquier PL, Paul L, TranDuy K. Surgical navigation in paediatric orthopaedics. EFORT Open Rev. 2016 May;1(5):152–159.
- Haglin JM, Eltorai AE, Gil JA, et al. Patient-Specific Orthopaedic Implants. Orthop Surg. 2016 Nov;8(4):417–424.
- Lin Y, Cai W, Xu B, et al. Patient-Specific or Conventional Instrumentations: A Meta-analysis of Randomized Controlled Trials. Biomed Res Int. 2020 2020:2164371.
- Teeter MG, Marsh JD, Howard JL, et al. A randomized controlled trial investigating the value of patient-specific instrumentation for total knee arthroplasty in the Canadian healthcare system. Bone Joint J. 2019 May;101-b(5):565–572.
- Lachiewicz PF, Henderson RA. Patient-specific instruments for total knee arthroplasty. J Am Acad Orthop Surg. 2013 Sep;21(9):513–518.
- Rivière C, Lazennec JY, Van Der Straeten C, et al. The influence of spine-hip relations on total hip replacement: A systematic review. Orthop Traumatol Surg Res. 2017 Jun;103(4):559–568.
- Corin Group. OPS: Optimised Positioning System. Corin Group. https://www.coringroup.com/uk/solutions/optimized-positioning-system-ops/. Published 2020. Accessed April 19, 2020.
- Florida Medical Clinic. OPS-Optimized Positioning System. https://www.floridamedicalclinic.com/fmchipdoctor/ops-optimized-positioning-system/. Published 2020. Accessed April 19, 2020.
- Good Design Australia. OPTIMIZED POSITIONING SYSTEM OPS™. https://good-design.org/projects/optimized-positioning-system-ops/. Published 2015. Accessed April 19, 2020.
- Shimmin A, Pierrepont J, Bare J, et al. Early results of the CORIN optimized positioning system: A registry analysis of 841 consecutive total hip arthroplasties. Orthopaedic Proceedings. 2019;102-B(Supp_1). https://online.boneandjoint.org.uk/doi/abs/10.1302/1358-992X.2020.1.083. Published February 5, 2020. Accessed April 25, 2020.
- Bartlett JD, Lawrence JE, Stewart ME, et al. Does virtual reality simulation have a role in training trauma and orthopaedic surgeons? Bone Joint J. 2018 May 1;100-b(5):559–565.
- Collins JP. International consensus statement on surgical education and training in an era of reduced working hours. Surgeon. 2011 9 Suppl 1:S3–5.
- Sevenoaks H, Ajwani S, Hujazi I, et al. Shift working reduces operative experience for trauma and orthopaedic higher surgical trainees: a UK multicentre study. Ann R Coll Surg Engl. 2019 Mar;101(3):197–202.
- Logishetty K, Rudran B, Cobb JP. Virtual reality training improves trainee performance in total hip arthroplasty: a randomized controlled trial. Bone Joint J. 2019 Dec;101-b(12):1585–1592.
- Wikipedia. Augmented reality. https://en.wikipedia.org/wiki/Augmented_reality. Published 2020. Updated April 18, 2020. Accessed April 19, 2020.
- Jud L, Fotouhi J, Andronic O, et al. Applicability of augmented reality in orthopedic surgery - A systematic review. BMC Musculoskelet Disord. 2020 Feb 15;21(1):103.
- Vadalà G, De Salvatore S, Ambrosio L, et al. Robotic Spine Surgery and Augmented Reality Systems: A State of the Art. Neurospine. 2020 Mar;17(1):88–100.
- Verhey JT, Haglin JM, Verhey EM, et al. Virtual, augmented, and mixed reality applications in orthopedic surgery. Int J Med Robot. 2020 Apr;16(2):e2067.
- Miyake RK, Zeman HD, Duarte FH, et al. Vein imaging: a new method of near infrared imaging, where a processed image is projected onto the skin for the enhancement of vein treatment. Dermatol Surg. 2006 Aug;32(8):1031–1038.
- Rojas-Muñoz E, Cabrera ME, Lin C, et al. The System for Telementoring with Augmented Reality (STAR): A head-mounted display to improve surgical coaching and confidence in remote areas. Surgery. 2020 Apr;167(4):724–731.
- Zarrinpar A, Lee DK, Silva A, et al. Individualizing liver transplant immunosuppression using a phenotypic personalized medicine platform. Sci Transl Med. 2016 Apr 6;8(333):333ra349.
- Koo CW, Anand V, Girvin F, et al. Improved efficiency of CT interpretation using an automated lung nodule matching program. AJR Am J Roentgenol. 2012 Jul;199(1):91–95.
- Clemens DL, Lee BY, Silva A, et al. Artificial intelligence enabled parabolic response surface platform identifies ultra-rapid near-universal TB drug treatment regimens comprising approved drugs. PLoS One. 2019 14(5):e0215607.
- Shademan A, Decker RS, Opfermann JD, et al. Supervised autonomous robotic soft tissue surgery. Sci Transl Med. 2016 May 4;8(337):337ra364.
- Esteva A, Kuprel B, Novoa RA, et al. Dermatologist-level classification of skin cancer with deep neural networks. Nature. 2017 Feb 2;542(7639):115–118.
- Lonner J. Robotics in Knee and Hip Arthroplasty: Current Concepts, Techniques and Emerging Uses. Springer Nature Switzerland AG; 2019.
- Martinez D. 5 Reasons Why Medical Grade Computers Matter in the Hospital. TechnologyAdvice. https://technologyadvice.com/blog/healthcare/medical-grade-computers/. Published March 22, 2018. Accessed April 10, 2020.
- Andrews S. Robot means “serfdom” in Czech and Asimov was the first to use the word “robotics”. The Vintage News. https://www.thevintagenews.com/2017/01/04/robot-means-serfdom-in-czech-and-asimov-was-the-first-to-use-the-word-robotics/. Published 2017. Updated January 4, 2017. Accessed April 10, 2020.
- Ranev D, Teixeira J. History of Computer-Assisted Surgery. Surg Clin North Am. 2020 Apr;100(2):209–218.
- Lechky O. World's first surgical robot in B.C. The Medical Post. November 12, 1985.
- Rahman J, Al-Tawil K, Khan W. Use of Robotic-Assisted Surgery in Orthopedics. In: Iyer K, Khan W, eds. General Principles of Orthopedics and Trauma: Springer; 2019.
- Hillel AT, Kapoor A, Simaan N, et al. Applications of robotics for laryngeal surgery. Otolaryngol Clin North Am. 2008 Aug;41(4):781–791, vii.
- Kyberd P. Technology: Robodoc carves a place in medical history. NewScientist. https://www.newscientist.com/article/mg13618492-600-technology-robodoc-carves-a-place-in-medical-history/. Published November 28, 1992. Accessed April 10, 2020.
- Bargar WL, Bauer A, Borner M. Primary and revision total hip replacement using the Robodoc system. Clin Orthop Relat Res. 1998 Sep;(354):82–91.
- Liow MHL, Chin PL, Pang HN, et al. THINK surgical TSolution-One((R)) (Robodoc) total knee arthroplasty. SICOT J. 2017 3:63.
- Yamamura M, Nakamura N, Miki H, et al. Cement Removal from the Femur Using the ROBODOC System in Revision Total Hip Arthroplasty. Adv Orthop. 2013 2013:347358.
- Wikipedia. da Vinci Surgical System. https://en.wikipedia.org/wiki/Da_Vinci_Surgical_System. Published 2020. Updated April 16, 2020. Accessed April 19, 2020.